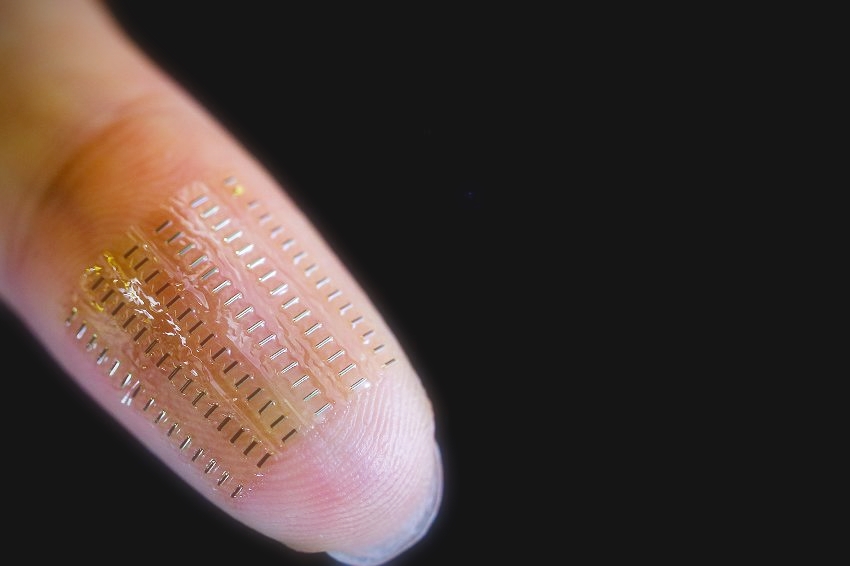
Chemnitz (Germany) The miniaturization of microelectronic sensors, microelectronic robots and intravascular implants is advancing rapidly. One challenge is the development of tiny but efficient energy storage devices that enable the operation of autonomously operating microsystems. In addition, these energy stores must be biocompatible in order to be used in the body. Now there is a first prototype that combines these essential properties.
The breakthrough came from an international research team led by Oliver G. Schmidt at the Technical University of Chemnitz. The researchers developed the smallest organic supercapacitor to date, which already works in artificial blood vessels and can be used as an energy source for a tiny sensor system to measure the pH value.
The currently smallest such energy storage devices are larger than three cubic millimeters. Oliver Schmidt’s team has now succeeded in producing a tubular biosupercapacitor 3,000 times smaller, which, with a volume of one nanoliter, takes up less space than a speck of dust and still supplies a voltage of up to 1.6 volts. The flexible tubular geometry of the nano-biosupercapacitor offers efficient self-protection against deformations caused by pulsating blood or muscle contraction. At full capacity, the presented power storage unit can operate a complex, fully integrated sensor system for measuring the pH value in the blood.
The researchers used origami technology for production, in which the materials required for the components are placed under high mechanical tension on a wafer surface. If the material layers are then detached from the surface in a controlled manner, the tension energy is released and the layers wind themselves up into compact 3D components with high accuracy and yield. The nano-biosupercapacitors produced in this way were tested in three electrolytes: saline, blood plasma and blood. Energy storage was sufficient in all three electrolytes, albeit with different efficiencies.
In the blood, the supercapacitor showed an excellent service life and held up to seventy percent of the initial capacity even after 16 hours. A proton exchange separator was used to suppress the rapid self-discharge. The team then examined the efficiency of the power storage in microfluidic channels in order to mimic blood vessels of various sizes. In these channels, the researchers simulated and tested the behavior of their energy storage devices under various flow and pressure conditions.
They found that the supercapacitors can provide their performance well and stably under physiologically relevant conditions in order to supply a pH sensor. To do this, the team integrated a pH-sensitive supercapacitor in a five-stage ring oscillator. This resulted in a change in the output frequency depending on the pH value of the electrolyte. This pH-sensitive ring oscillator was brought into a tubular 3D geometry, so that a fully integrated and ultra-compact system of energy storage and sensor could be created. The hollow inner core of this tiny sensor system serves as a channel for the blood plasma.
The original publication with more details can be found here: https://www.nature.com/articles/s41467-021-24863-6